There are many real-world scenarios where mobility is needed. Interdisciplinary teams at Michigan Tech tackle research challenges in the air, on land, and even underwater.
Air
Everyone's heard of drones. Some of the press has been sinister. But these unmanned flying machines, operating autonomously and by remote control, can go where people can't or prefer not to venture—under bridges, inside culverts, down deep and narrow shafts—carrying sensors that transmit information about the conditions and critical needs of those systems.
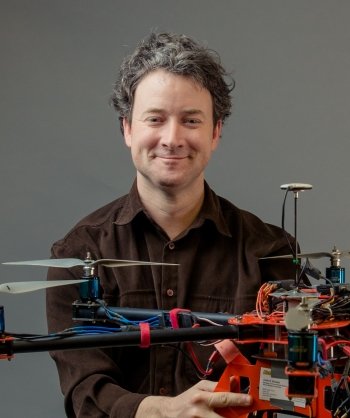
Colin Brooks, a senior research scientist at the Michigan Tech Research Institute (MTRI), leads the University's work using drones or unmanned aerial vehicles (UAVs) to help federal and state agencies develop safer, more effective, lower-cost ways to monitor transportation infrastructure.
Using high-resolution aerial imagery to understand conditions on the ground is nothing new, Brooks points out. "The oldest surviving aerial photo is of Boston from 1860, taken from a tethered balloon," he explains. "We're just making data gathering quicker, easier, safer, and more detailed for rapidly understanding our transportation infrastructure."
Brooks, who specializes in remote sensing technology and geographic information systems (GIS), heads Tech's UAV transportation research team, which includes Rick Dobson, Thomas Oommen, Tim Havens, Tess Ahlborn, Kuilin Zhang, and Amlan Mukherjee.
MTRI deploys five main UAVs: Bergen hexacopters, a heavier-lift Bergen quad-8 system, a DJI Mavic Pro, a DJI Phantom 3 Advanced, and a Mariner 2 Splash waterproof system. The hexacopter platform is used primarily to collect high-resolution 3-D optical and thermal data of bridge decks, construction sites, and road corridors. The larger Bergen quad-8 system, with four pairs of rotors, is used to carry larger sensors and sensors in combination. The Mariner 2 Splash can land and take off from the water, helping inspect the underside of bridges over water. The Mavic Pro and Phantom 3A are smaller quadcopter systems. Both are useful for obtaining traffic operations data, imaging hard-to-reach locations, and providing site documentation photos.
Recently, the Michigan Tech team incorporated UAV technologies into the Michigan Department of Transportation's (MDOT) day-to-day operations. Five bridges, three road corridors, and one construction site were analyzed, producing seven well-documented standardized geospatial outputs based on UAV imagery. The team showed MDOT how to use optical, thermal, and LiDAR sensors for corridor evaluations, bridge condition assessment, and traffic operations data analysis. A detailed cost-benefit analysis demonstrated that UAVs can provide better estimates of distress, leading to lower maintenance costs.
Up to now, agencies responsible for roads have been reactive, checking out problems after someone calls to complain, Brooks explains. "This technology turns reactive responses to proactive responses."
Brooks also led the Michigan Tech components of two new UAV transportation research efforts. Working with the University of Texas at Arlington on a Texas Department of Transportation project, Brooks' team analyzed rail crossing roughness, identified erosion areas along railways, and documented bridge fascia conditions using UAV-collected imagery. Working with the City University of New York under the Federal Highway Administration (FHWA), the team completed 3-D modeling of two bridges in eastern Maryland, demonstrating how UAV-enabled sensing combined with sonar sensing can help evaluate post-hazard scenarios where bridge components may have been affected by bridge scour or other issues.
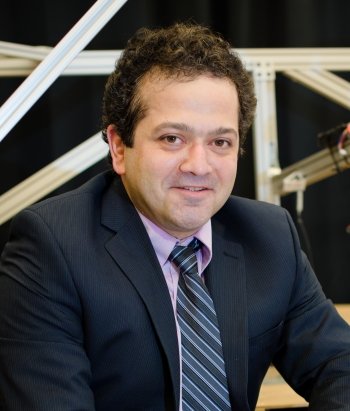
While Michigan Tech works to develop good uses for drones, University researchers also designed a way to get rid of bad ones: Mo Rastgaar's drone catcher, which captured the imagination of the media and the public.
It's a simple system: a launcher that shoots a big net attached to a large drone by a string. The system can be autonomous, controlled by a ground-based human pilot, or a combination of the two.
After an intruding drone is spotted, the drone catcher gives chase, firing the net from a distance of up to 40 feet. Because the net is so big and can be deployed so quickly, it can capture even the fastest, most maneuverable small drone.
"If the threat is a drone, you really don't want to shoot it down—it might contain explosives and blow up," Rastgaar, associate professor of mechanical engineering, explains. "What you want to do is catch it and get it out of there."
Rastgaar and his colleagues are working to get their drone catcher patented and commercialized.
Water
While at times the isolation, snow, and resulting travel can be frustrating for Michigan Tech researchers, the campus location and its arctic-like environment offer many benefits. This is particularly true for mobility research in and on the water.
The Great Lakes Research Center (GLRC) is water mobility's flagship. The GLRC does, literally, have ships—a fleet that includes several research and survey vessels, remotely operated vehicles (ROVs), autonomous underwater vehicles (AUVs), and, hopefully a new addition coming soon, an autonomous surface vehicle (ASV).
"Michigan Tech is ideally situated to help develop the State's vision for mobility in all sectors, notably in the marine sector," says Guy Meadows, the director the GLRC and Robbins Professor of Sustainable Marine Engineering. "Not only is Tech's depth and breadth substantial on the technology side, but we are also ideally located as a testbed for year-round autonomous marine systems. Marine traffic, both commercial and recreational, is far less here in central Lake Superior than most places in the world."
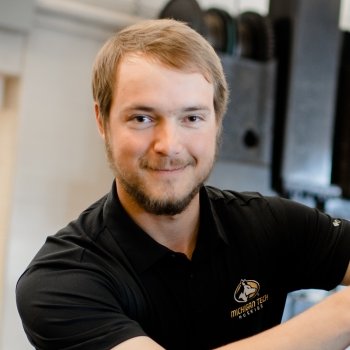
Travis White '11 is the backup captain for the GLRC's R/V Agassiz and also the latest research engineer hired at the GLRC; he focuses on the technology and operational environment behind the center's autonomy research and is actively working on the proposal to acquire an autonomous boat.
The sensors and control systems scale up, and boats can be classified at different levels of autonomy; the highest levels are able to analyze real-world scenarios and make decisions with minimal human input. An ASV can gather data on water quality, algae blooms, fish populations, and thermodynamics—zigzagging in research surveys for up to five days and 840 nautical miles, pushing into the shoulder seasons and difficult-to-reach areas. One challenge with ASVs, however, is unpredictable—rough water.
"The Keweenaw Waterway has the only US Coast Guard station for all of the Great Lakes that has these 47-foot surf boats designed to go out in over 15-foot seas," White says. "Anything we learn here about rough water in central Lake Superior can be applied to the Atlantic or Pacific or other oceans."
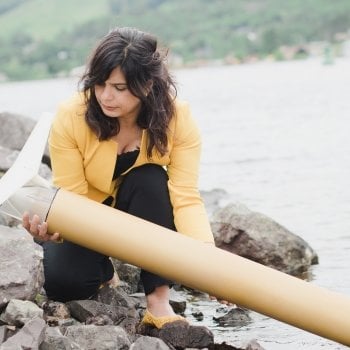
Below the waves is another challenge. For underwater mobility, the major obstacles are communication, positioning, and limited energy. That's why a marine robotics engineer and an underwater acoustics electrical engineer teamed up using reinforcement learning.
"Water is less forgivable," says Nina Mahmoudian, the Lou and Herbert Wacker Associate Professor in Autonomous Mobile Systems. "We want robots to learn from their mistakes."
To do so, Mahmoudian is partnering with Zhaohui Wang, assistant professor of electrical and computer engineering and a member of the GLRC. Together, they are working on a low-cost and high-modularity mobile network infrastructure including low-cost AUV and ASV systems connected underwater by soundwave-based communications. The goal is to study fundamental challenges to achieve seamless integration of acoustic communication modules within a fleet of operational vehicles.
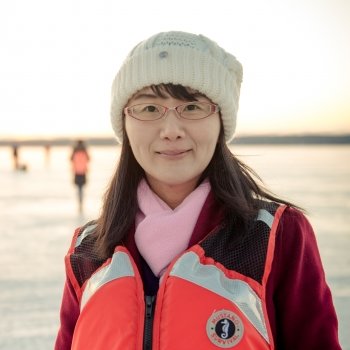
Coordination, of course, is all about collaboration. On a policy scale, the GLRC is leading the charge on the economics and governance through the Smart Ships Coalition, a Michigan initiative to inform the development for rules of the road for autonomous vessels in the Great Lakes. Ultimately, the US Coast Guard will be responsible for implementing and enforcing future regulations on autonomous vessels for commercial and recreational use.
"We have to solve a lot of challenges in order to set baseline rules of conduct for autonomous boats," White says, adding a recent parallel can be found in adoption and commercial use of drones, which now requires a drone pilot license. "That's where I see the GLRC making a difference, to establish a framework and facilitate those conversations."
Ground
Rush-hour traffic. Semi-trucks change lanes. Off-ramp construction reroutes travel. Cars line the streets, buses pull away from stops. Pedestrians are everywhere. Rain starts to fall, the jolt of crossing a railroad—a sudden stop as a car runs a red light.
In the organized chaos of modern ground transportation, what mobility technology will pass the test of real-life scenarios?
Let's start with a single car—and let's go all out to see what the not-so-distant future holds. The blue 2017 Chevrolet Volt looks familiar, but it's different inside and out.
"The Volt has one of the most complex drive systems of any vehicle today—with an internal combustion engine and two electrical motors," says Jeff Naber, director of the Advanced Power Systems Labs (APS LABS), adding that his team will equip the car with sensors, software, and a few other improvements. "These seamless technologies will operate without the driver being aware of what's happening under the hood."
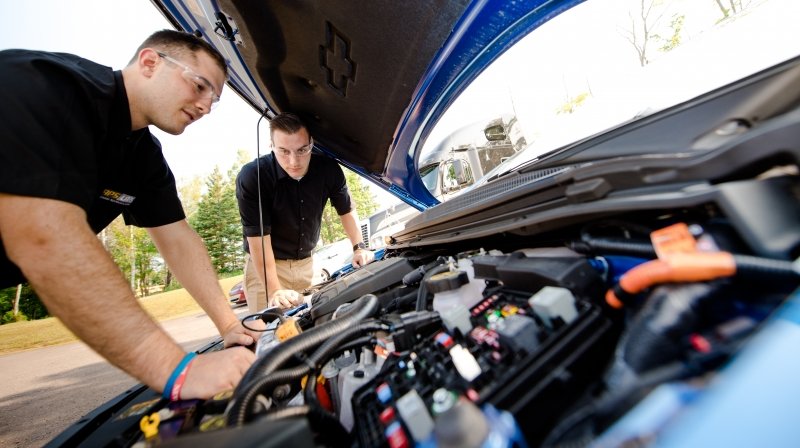
The Advanced Power Systems Labs work with a fleet of Chevy Volts to push autonomous and electric vehicle technology to the next level.
The car is one of eight in the fleet at APS LABS and is part of a project funded by the US Department of Energy's ARPA-E NEXTCAR program that seeks to reduce fuel consumption and increase electrical range through connected and automated technologies. The APS LABS team has worked with other university engineers and scientists, demonstrating that optimized vehicle powertrain control, enabled with vehicle connectivity, can reduce fuel energy by more than 20 percent and increase the electric vehicle range by more than 6 percent.
Fuel itself—and injecting it into an engine— is another aspect of mobility research. Xiucheng (Sheldon) Zhu '16, a mechanical engineering graduate student, works in the Alternative Energy Research Building, a nondescript brick facility in Hancock. Inside, the equipment is anything but nondescript. Under his advisor, Seong-Young Lee, Zhu takes simulations and watches them come to life in a 1.1 liter optically accessible, constant-volume combustion chamber. This rare device is used to study ignition, fuel injection, along with spray and emissions. Researchers can see the entire combustion process through a four-inch sapphire window.
"Few people have a chance to work with this type of equipment," Zhu says. "There are only two of these combustion vessels in the US, one here and one at Sandia National Laboratory."
Once fired up and moving, researchers need to understand how a car navigates traffic. Intervehicle communication software is the key (see Mobility: Sensors below). This can be vehicle-to-vehicle communication ("Hey! I'm driving here"), vehicle-to-infrastructure communication ("Roadwork on the bridge"), and vehicle-to-anything communication ("Accident ahead" or "I'm a bicycle" or "Cellphone alert: detour"). The three are respectively called V2V, V2I and V2X technology; they all rely on cloud computing to work.
But could a car designed to navigate Detroit during rush hour handle a Keweenaw blizzard? The Keweenaw Research Center is ready to find out. They have more than 100 lane miles in four snow-packed handling loops, circle tracks, vehicle dynamics surfaces (locally known as "ice"), slopes ranging from 15 percent to 40 percent, and a big cold room that dips down to 40 below.
This kind of engineering is what Jeremy Bos, assistant professor of electrical and computer engineering, calls "autonomy at the ends of the Earth." Rather than bemoaning Keweenaw winters, Bos wants Michigan Tech to embrace them—and push mobility research outside the yellow lines in a full blizzard of off-road and real-world possibilities.
"I see a renaissance in the state driven by new mobility technologies, which leverages the considerable engineering talent that already exists here," says Dan Fuhrmann, chair of the Department of Electrical and Computer Engineering. "Engineers who can cross disciplinary boundaries among mechanical engineering, electrical engineering, and computer science are needed to keep this movement vital."
Sensors
Spanning air, water, and land—sensors make the mobility world go around. They are the eyes, ears, nerves, and guts of mobility technology, but they're only as effective as the communication between systems.
Several researchers from the Department of Electrical and Computer Engineering are working on the science, technology, engineering, and math behind sensors.
Connected Controls
Sensors within a vehicle can be enhanced. A member of the NEXTCAR research team, Bo Chen focuses on developing a model predictive control system to optimize a hybrid vehicle's powertrain. The goal is to maximize energy and fuel when switching between the car's electric motors and internal combustion engine. Based on input from vehicle velocity, power trajectory, traffic simulation, road grade, and many other factors, the most efficient powertrain control can be maintained.
Comm Networks
Sensors feed systems that require connection to function. That communication has to happen inside and outside a vehicle. Aurenice Oliveira works at the interface of the two domains and she says the emerging technology has the power to reduce vehicle crashes by 80 percent. To improve safety, traffic efficiency, and fuel consumption all around, Oliveira works within the Vehicular Ad Hoc Network (VANET) to meet the biggest communication challenges—poor connectivity and the fact that cars are moving targets.
Platform Fusion
The sum is more than its parts. Tim Havens wants to help produce a total combined measurement that is more than adding up data from individual sensors. A fusion platform that integrates multiple different types of sensors, which all complement and round out how they sense the world together, will make real-time analysis more feasible. That's crucial for detecting explosive devices on battlefields, unexpected road obstacles, and weaknesses in bridges.
Michigan Technological University is a public research university founded in 1885 in Houghton, Michigan, and is home to more than 7,000 students from 55 countries around the world. Consistently ranked among the best universities in the country for return on investment, Michigan’s flagship technological university offers more than 120 undergraduate and graduate degree programs in science and technology, engineering, computing, forestry, business and economics, health professions, humanities, mathematics, social sciences, and the arts. The rural campus is situated just miles from Lake Superior in Michigan's Upper Peninsula, offering year-round opportunities for outdoor adventure.