Water and oil don't usually mix, but these interdisciplinary researchers at the Great Lakes Research Center have answers when they do.
Resting on a cork stand, the flask sits high on a shelf in Guy Meadows' office. Meadows, director of the Great Lakes Research Center (GLRC) at Michigan Tech, reaches up and pulls the flask down. The contents slosh; a narrow piece of pen cap surfs in the middle, trapped at the interface. With a delicate swirl, Meadows gets a small wave moving, much like the slop of water in a bathtub, several emulsified bubbles clinging to the tipping oil and water surface.
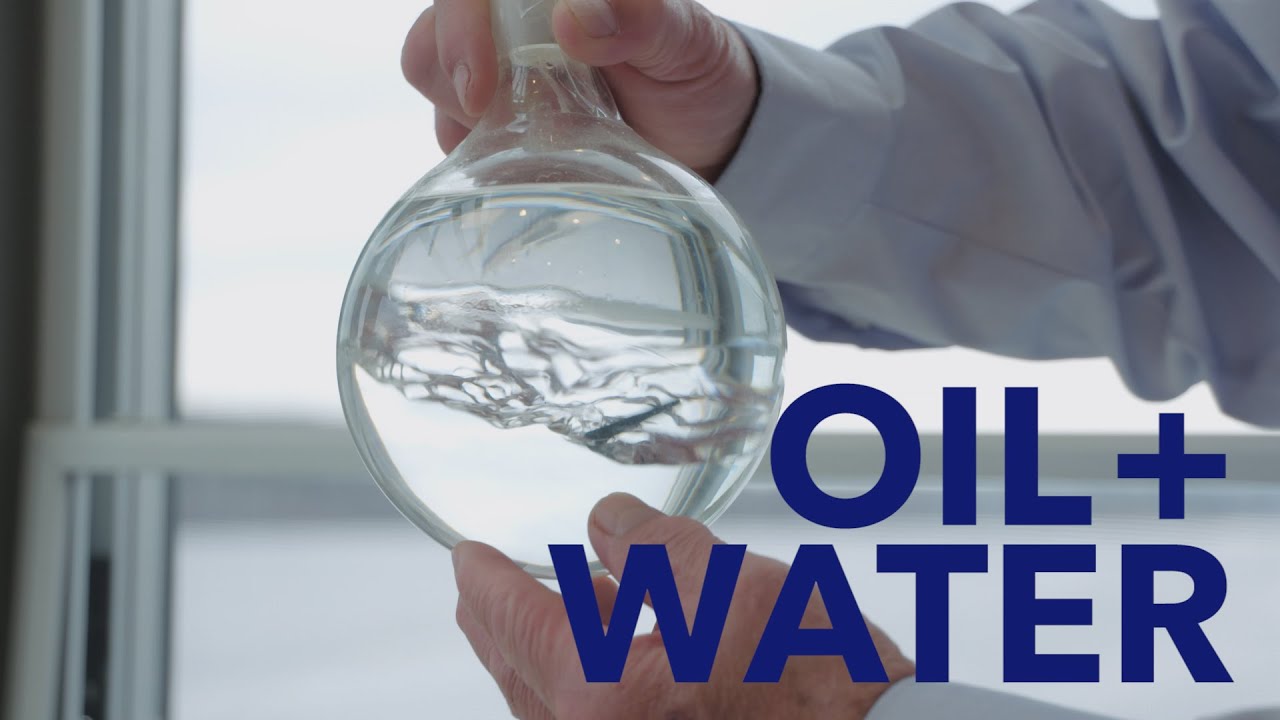
Storm in a Bottle
Contained in a flask, the movement is hypnotizing—beautiful even. But in the environment, we try very hard to keep oil and water separate. To date, the majority of oil spill research focuses on saltwater environments and little has been done in freshwater. Scientists at the GLRC are remedying that. Using high-tech equipment and extensive fieldwork, they are working to help prevent spills, monitor and assess at-risk locations, predict spill patterns with supercomputer models, and understand oil-eating microbial communities across the Great Lakes.
Under the Surface
Oil floats on water. Usually. But as the complexity of the 2010 Deepwater Horizon spill in the Gulf of Mexico showed researchers, understanding what happens below the waves is crucial to mitigating an oil spill.

Meadows uses the water-oil flask from his office, which he calls his ocean in a bottle, to explain underwater complexity. Specifically, how layers interact in stratified waters. As he swishes the flask, the oil and water interface in the center roils and storms. The top remains perfectly still.
"Currents and layers below the surface can be very complex," Meadows says, explaining that a better understanding of these dynamics can help predict how oil would migrate in water. "This is particularly important in places like the Straits of Mackinac where two large bodies of water are contending for equilibrium with each other—it creates complicated and constantly shifting current patterns."
Flows within the Straits could greatly affect a spill and what shores might be impacted. Meadows heads up a team at the GLRC that monitors these currents along with several field sites. They use buoys, remote operated vehicles (ROVs), autonomous underwater vehicles (AUVs), and sonar. The field observations are complemented—and even driven by—cutting-edge modeling using the in-house supercomputer Superior.
Modeling Motion
For the GLRC team, work by Pengfei Xue, an assistant professor of civil and environmental engineering, is the research's keystone. He studies coupled hydrodynamic and climate models to provide insight into potential oil spills.
"Basically, we are trying to understand the movement of microparticles in water," Xue explains. "If we look at continuous and varying water flow patterns through space and in time, then we can predict where the particles will move."
The basis of hydrodynamic models is the set of mathematical equations that describe the motion of fluids with the conservation of water mass and momentum and energy. Using these numerical models, which are so intricate they require a supercomputer to run, Xue can address complex problems like oil spills.
To start, Xue inputs data on lake shoreline, water depth, wind field, wave height, temperature, and many other variables in the model. Then he makes meaning of the raw information using the Superior supercomputer and provides a 3-D simulation of water flow conditions. The end result is a visualization of the relationships of water flow, thermal structure, and wind, showing impacted area, duration, and strength if oil would disperse through an area. It's like a pointillist painting: the individual dots and data blur together, revealing a whole picture.
Since models are approximate mathematical descriptions of real phenomena, though, the challenge is getting enough dots of just the right color with just the right placement to blend into a realistic whole.

"Observational data is usually intermittent," Xue says. "Modeling helps us develop continuous data points to get a clearer picture of what's happening.
"Xue's hydrodynamic model for the Great Lakes is detailed. So detailed that it is more like a pixelated digital photograph than a pointillist painting. It adopts an unstructured-grid finite volume approach and has the capability of resolving the lakes' geometrical complexities with sufficient detail to determine the flow conditions at previously unprecedented resolution both horizontally and vertically. This level of hydrodynamic predictive capacity is critical for understanding hydrodynamics and its impact on our ecosystem.
In addition to microparticle modeling, another part of the GLRC's work focuses on the microscale.
Oil-Eating Microbes
At the interface of oil and water, a bunch of microbes serve up an unlikely meal. In an oil spill, bioremediation calls for the Alcanivorax, Neptunmonas, Oleispiras, and Oceanospirillales groups—except there aren't any of these ocean-dwellers in the Great Lakes. Biologist Steve Techtmann is on a mission to find local, freshwater oil-eating microbes and understand their preferred environments.
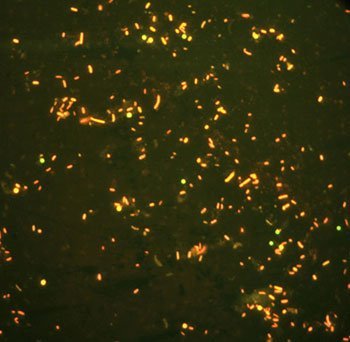
"We need an ecosystem view on clean-up and mitigating oil spills is really an exercise in microbial ecology," Techtmann says, adding that knowing the type of oil involved, proximity to shore, current patterns, nutrient limitations as well as seasonal variations can all impact microbes' effectiveness.
However, he says there is no "magical spray" of oil-chompers that miraculously clears up a spill. Knowing how many oil-eating microbes are naturally present, how they interact with oil, and their potential side effects are crucial for successful mitigation.
"Oil is a complex contaminant—that's where the biology comes in," Techtmann says, adding that these microbes are difficult to grow and study in the lab. "Since no one has really looked at the freshwater species, we have the chance to figure out who are the dominant players in these systems."
Again, Xue's models can help predict and provide insight into the most likely locations of these oil-eating microbial communities. And the models depend on data validated by a vast network of buoys in the Great Lakes.
Big Data
The buoys are bright yellow, about 16-feet tall from base to the top wind gauge, weigh about 600 pounds, and can manage 30-foot waves. These big buoys provide big data.
"We have more than 30 years of data and we are starting to see some preliminary trends," says Caryn Murray, an undergraduate research assistant who has spent the past year crunching data from the Great Lakes Research Center buoys as well as National Oceanic and Atmospheric Administration buoys. "The experience has taught me the value of having lots and lots of data, covering many years.

Murray is digging back into buoy data starting in the 1980s, focusing on Lake Superior. Today, each buoy makes a cell phone call every 10 minutes, relaying data from May to October.
One particular buoy, deployed in the Straits of Mackinac, is a key data collector. In partnership with energy company Enbridge, the buoy monitors water quality and conditions in the Straits to provide safety alerts for boaters, environmental data, and to monitors the region near Enbridge's Line 5 pipeline. Below water, the collaboration continues with bottom sonar surveys of the pipeline and surrounding terrain. Companies are required to do pipeline inspections at least once every five years; with the buoys, ROVs, and AUVs, Enbridge and the GLRC team, led by Meadows, are able to gather observations every month.
"Any type of infrastructure needs monitoring," Meadows says. "No one wants an oil spill, but we need to prepare for a worse-case scenario—and we're leading the research to mitigate that risk."
Michigan Technological University is a public research university founded in 1885 in Houghton, Michigan, and is home to more than 7,000 students from 55 countries around the world. Consistently ranked among the best universities in the country for return on investment, Michigan’s flagship technological university offers more than 120 undergraduate and graduate degree programs in science and technology, engineering, computing, forestry, business and economics, health professions, humanities, mathematics, social sciences, and the arts. The rural campus is situated just miles from Lake Superior in Michigan's Upper Peninsula, offering year-round opportunities for outdoor adventure.